Science Tribune - Article - October 1997
http://www.tribunes.com/tribune/art97/mari.htm
Measuring the colours we perceive
Daniele Marini and Ludovica Marini
Dipartimento di Scienze della Informazione Universita degli Studi di Milano Via Comelico 39, 20135 Milano, Italy. Fax +39+2 55006 334
Website : http://hpux.dsi.unimi.it/imaging/retinex.html
E-mail : marini@eidomatica.dsi.unimi.it.
(a) (b)
Differences may exist between the physical attributes of objects, such as colour, measured by instruments and what we humans actually perceive. It would be helpful in several fields - as in medical imagery - to be able to resolve these differences by having a model that reconstructs our subjective vision of things.
It was recognized early on that colours are in the mind's eye. The Greeks considered colour perception to be a subjective experience and this view crosses the ages with, however, several interludes stressing the more objective aspects (e.g. during the Renaissance or when Newton formulated his optical theory ...).
Several descriptive models of colour perception have been elaborated over the years (the tri-stimulus model, Hering's model, the zonal modal of Müller and Helson-Judd). In the late 1960's Edwin Land proposed a theory - the Retinex theory (from Retin(and cort)ex) - which assumes that colour perception depends strictly on the neural structure of the human visual system. The theory has given rise to a mathematical model for the subjective nature of colour perception. In this model, the relative reflectance of a coloured area is given by the mean reflectance of random paths leading to that area. We propose that a suitable random path is the one given by Brownian motion because this fits in with our present knowledge of visual neurophysiology.
We shall illustrate by means of several images how this model can emulate human colour perception, in particular the perception of "colour illusions" and of colour constancy, and how it can be used to filter natural images and to equalize colour and lightness.
________________________________________________________________________________
In the perception of colours, do our eyes deceive us or should we be able to reproduce and measure what we actually see ? Are humans subject to colour illusions ?
"Colour illusions" and colour constancy
So-called "colour illusions" occur when an observer experiences colour stimuli that do not correspond to the actual physical effects deriving from the interaction between the object and the light that impinges upon it. A small grey rectangle placed against a large red background appears light green; when placed against a green background, it appears light red, that is, in each case, the colour that is opposite to the background colour (1). In Figure 1, the top and bottom brown rectangles in the image on the left appear different although the saturation values are the same (S = 97). In the image on the right, their saturation appears higher when it is in fact lower. Here again, the "illusion" arises from the contrast between the object and its surroundings and is experienced by any observer who is not colour-blind.
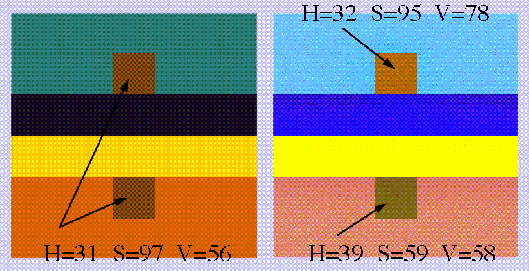
Fig.1. Albers' colour illusion ( H = hue, S = saturation, V = value or brightness)
A different situation arises with colour constancy. Our perception of colours is normally independent of the characteristics of the light source - at least within a given range - whereas the physical world (chemical photography and computer-based image acquisition) reacts non-uniformly to changes in light conditions. As any photographer knows, pictures taken in warm artificial light and not sunlight have an orange-red dominant colour that pervades all the objects making up the scene. The TV monitor looks black even though its natural colour is dark brown-grey.
Perceived colour : a product of the interaction between the world and us
Maybe, however, we are not even subject to "colour illusions". As Hardin says (2), colours may not be an attribute of the "external" world but what we actually perceive, the product of an interaction between us and the world.
Characterising such an interaction is a complex business. It might have something to do with Husserl's notion of "intentionality" (3) and with the way human perception is structured, but it also has a biological foundation in the feedback systems present in the brain cortex. In fact, more than one area of the brain cortex processes light stimuli and gives rise to a sensation of colour (c). We do not know, however, how these different areas interact to provide a unified vision and a consciousness of the perceived stimulus.
A short history of subjectivity in colour perception
The notion of subjectivity in perception - and in visual perception in particular - dates back to the Greek philosophers - Empedocles for one (4) - for whom vision was an activity of the human eye. The movement of an interior light toward the exterior was thought to produce an image of the world: "Sunlight is not enough for the vision process, light emanating from the body is also necessary ". Plato (5) endorsed Empedocles' "vision from within" but also interpreted vision metaphorically, as knowledge in general. For Democrites (6), the qualities of objects sprang from an encounter between the quantitative properties that emanate from them and our organs of perception. In all these theories, visual perception is explained by movement, and vision is a process of the soul. Aristotle (7) used a biological basis to describe perception and made psychology a science for the first time. For him, visual perception is due to an action on - and to the activity of - the eyes.
Middle Age and Renaissance philosophy tried to objectify and quantify visual perception and put little emphasis on subjectivity. However when, centuries later, Descartes (8) observed retinal image inversion through a bull's eye, he was unable to justify perception as a "res extensa" and introduced a spiritual principle "res cogitans", an immaterial mind which uses human sensors to observe the world.
Goethe (9) fiercely contested Newton's optics theory (10) (d) and its focus on physical properties. He said that colours arise in the eye and based his description of their properties on optical phenomena he himself observed. For Goethe, colours were analogous to perspective and proportions, i.e. formal categories that we process to make the observed world conform to the internal order of our brain. Light is needed to see colours because they are not part of nature but a product of our mind and eyes.
Heisenberg (11) tried to reconcile Goethe's and Newton's views. He suggested that the cartesian dichotomy between an objective and a subjective world - each with its own validity - would be resolved by the study of the neurological system which would ultimately be described in terms of mathematical structures.
Contemporary researchers such as Sacks, Hubel and Seki, besides focussing on a physiological approach as predicted by Heisenberg, also emphasize psychology. From an analysis of clinical cases, Sacks (12) identifies areas of the brain cortex with a role in colour perception. He also stresses the role of memory, emotions, and culture and relates colour perception to a holistic human experience.
Descriptive models of colour perception
If we assume that there is an interaction between the outside world and ourselves, we can adopt a suitable methodology to study colour perception and
(i) perform an objective study of the physical object-light interactions
(ii) develop quantitative models to investigate the psycho-physical aspects of colour perception (e).
The spectral composition of light is the physical basis for the formation of colours. The discovery in the mid-1800's of lightwave-sensitive cones in the human retina gave rise to the tri-stimulus theory according to which signals generated by three sensors are transmitted to the brain where the sensation of colour arises. This theory, however, does not solve the problem of colour constancy because, as Helmholtz (13) says, it is ".. discounting the illuminant". The colour is judged by inference. Present day tri-stimulus theory characterizes the spectral response Giof each sensor as a function of a sensitivity gi and of an energy distribution function of illumination Ei
Gi = f(giEilambda), where lambda is the light wavelength.
A colour can be modelled as a combination of the responses of the three sensors to any given stimulus.
Hering's theory (14) is based on six different colour sensations generated by opposing neural signals. They are grouped into three pairs (white/black, yellow/blue and red/green) which correspond to the two-fold, opposing actions of light on three retinal substances : a "catabolic" action produces a sensation of white or yellow or red, an "anabolic" action a sensation of black, blue or green. An antipodal colour coding process does exist in the retinal neural network structure and has led to the so-called zonal theory which has been used to support observations on colour conceptualisation. For instance, we can name impossible colours - "greenish red", "bluish yellow" - although whether people able to conceptualise such colours actually exist is a moot point.
The zonal theory of Müller and Helson-Judd (15) combines some of Helmholtz' and Hering's best ideas. Müller assumed that colour vision can be decomposed into at least three stages. In the first stage, the light stimulus is absorbed and coded into electrical signals according to the tri-stimulus model; in the second and third stages, the signals are recoded in a neural network to produce three new signals, one achromatic signal and two antagonistic chromatic signals that account for the antipodal colour theory of Hering. Judd developed a mathematical model for this process and used it to solve the colour constancy problem. His model predicts perceived colour quite accurately but without any explanation.
All these three mathematical models use geometric (Euclidean) space where each point, given by three co-ordinates X, Y, Z, identifies a colour. This metric can be used to measure colour distance and to quantify any hue but, like RGB co-ordinates (f), produces colour scales that are not smooth to the human eye. Munsell (15) has proposed a new colour space more in harmony with our vision that is now extensively used in colour design whereas the CIE (Commission international de l'éclairage) has elaborated two other models (CIELuv and CIELab) (15) - transformations of the X,Y, Z colour spaces - that provide a mathematically sound basis for the human notion of distance between two colours.
Land's Retinex theory
The above theories confine colour vision to the retina or, in the case of Judd, to an unspecified neural level. Marr (16) considers them descriptive models and not theories. For him, the only real theory is that of Edwin Land - the Retinex theory (17) (18) - which tries to integrate what happens at the level of the retina and brain cortex within the neural structure of the human visual system. Not knowing whether the retina or cortex has the key role, Land coined the term "Retinex" (retina and cortex ). The fundamental observation that drove Edwin Land to develop his theory was : "The eye, in determining colour, never perceives the extra red [produced by a tungsten lamp] because it does not depend on the flux of radiant energy reaching it."
Land's first experiment was based on the projection of two black and white slides, one through a red filter, the other through a green filter. Surprisingly, what appeared was an image with all the original colours ! The filters had in fact reproduced the spectral response of the cones of the human retina. He then went on to show that there is a parameter "lightness" associated with every object of a scene. Intuitively, we can think of lightness as a measure of relative clarity, of the brightness of a surface compared to surrounding surfaces. The lightness of an object is unaffected by the lighting conditions or by the object's location with respect to others of different lightness. We thus perceive lightness independently of the light flux that impinges upon our eye.
In a second experiment, Land and McCann showed that, by stimulating rods and high-wavelength (red)-sensitive cones with light, one can create the sensation of a full range of colours. This implies that colour perception does not depend on spectral fluxes reflected by single objects but on a comparison of lightness.
A third experiment demonstrated that rectangular patches of different colours (similar to the drawings of the painter Mondrian and therefore called Mondrian patches) produce a perception of colour that is independent of the reflected spectral flux, or rather of the product of spectral reflectivity by incident light energy produced by a light source, which is the property of colour constancy.
Land and McCann defined a measurable physical quantity which can be correlated to lightness. It is the ratio between the integrated radiance from a Mondrian area and the integrated radiance from a white paper measured under white illumination, using three filters whose spectral response is equal to the cone spectral sensitivity.
Fig. 2. Response curves of human retinal cones (short, medium and long wavelengths correspond to blue, green and red light, respectively)
Colour perception is produced by a comparison of the three resulting values even if, as Land says, one cannot identify corresponding neural regions in the human visual system. The three numbers characterise a measure of lightness and ultimately define a geometric space that can be used to measure and compare colours and colour scales.
Introducing Brownian motion into Land's theory
In the mathematical model derived from Land's theory, the relative reflectancy of a coloured patch is given by the mean value of the relative reflectancies computed along random paths to that patch (g). The result also depends on the threshold level chosen to minimize low reflectance ratios which correspond to smooth changes in colour due to non-uniform illumination. How to choose the random path ? We propose a solution based on Brownian motion because the centres of certain receptive fields in the brain cortex, in particular in area V4 (c), are distributed as a Brownian path (h). Indeed, the application of an approximate Brownian path to the Retinex algorithm has greatly improved its effectiveness and speed.
We cannot describe nature per se, without our own intervention and observation (i). The Retinex algorithm, with its Brownian approximation, thus fulfils the role of a non-interacting observer, abstracting the cognitive process from Husserl's intentionality. It provides us with an extremely useful heuristic tool for the study of nature. Once an image obtained with a light camera, digital camera, or scanner, is filtered by the Retinex algorithm, it is transformed into a picture that looks much more like what we actually see. In Albers' example, the position of the two brown squares on differently coloured backgrounds determines the colour seen (Fig. 1) but, once filtered by the Retinex algorithm, the squares do have different saturation values. Our model thus objectifies and quantifies the subjective faculty we have to distinguish between two colours which colorimetry tells us are the same.
Here are a few examples.
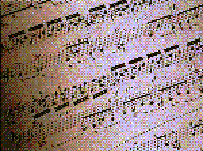
Fig. 3. Simulation of colour constancy. Left : Non-uniform illumination gives rise to a dominant orange colour. Right : Suppression of this dominant (Retinex algorithm with a 20-path brownian motion).
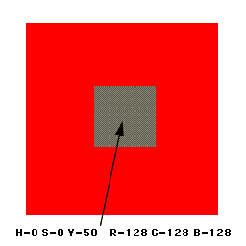
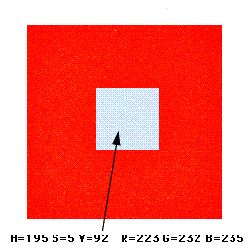
Fig. 4. A "colour illusion". Left : A small grey square placed against a red background appears greenish. Right : The colour of the square reconstituted by Retinex.
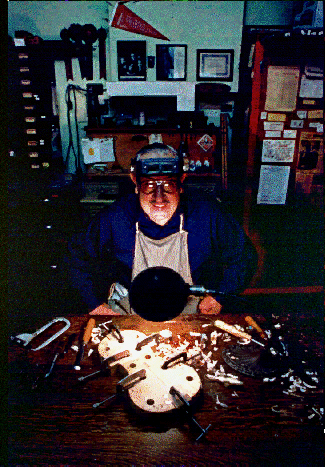
Fig. 5. Equalisation of colour and lightness. Note the detail of the background in the right panel. (Retinex algorithm with 40-path brownian motion).
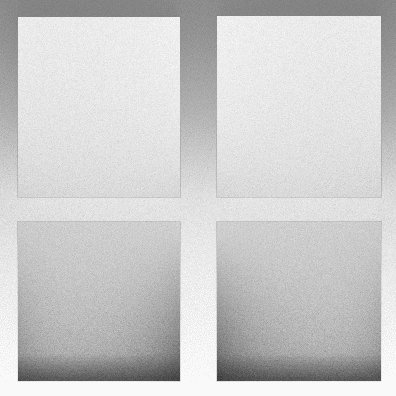
Fig. 6. An illusion by Joseph Albers. Left : The four left panels appear striated. Right : The background of the four right panels changes smoothly. (Retinex algorithm with 200-path Brownian motion).
For further examples, see [http://hpux.dsi.unimi.it/imaging/retinex.html /].(Click here)
The self-reference paradox
If the algorithm quantifies the sensation of colour and behaves as if it were an external observer, what happens when we observe the image produced by the algorithm, when we interact again with the system ? In fact, to keep the image free of subjective interference, we should analyse it using another computational simulation, and then analyze the next image by another simulation, and so on, endlessly. What is the meaning of this endless chain? It could indeed be empirical evidence drawn from computations that perception is the result of a subject-object interaction.
Notes
(a) Ludovica Marini is now at University College, Department of Anatomy and Developmental Biology - l.marini@ucl.ac.uk
(b) This article is based on a presentation at the "Trieste Symposium on Perception and Cognition" 1995. The work was partially financed under contract num. 95.02816.ST74, CNR Strategic Project . The authors are grateful to Alessandro Rizzi, who first implemented the Retinex algorithm in their unit, and to Roberto Rovetta who improved its efficiency.
(c) Here is a brief description of how the brain processes a coloured image: The retina is linked to the primary visual cortex (also called striate cortex V1) via a subcortical structure known as the Lateral Geniculate Nucleus (LGN). The retina is not at the origin of colour perception but produces wavelength stimuli that are processed by different areas of the brain cortex. These are primarily : V4, the parvocellular strata of the LGN, cellular "blobs" in V1, and subtle stripes in V2 (19) (20). The cellular "blobs" in V1 receive signals from wavelength-sensitive LGN cells but it is probably V4 that is active in selective wavelength processing. V4 responds to colour stimuli presented "in context" whereas V1 also responds under "void conditions", i.e. when the subject is isolated from any other stimulus. Thus, visual stimuli are selected on the basis of function and are processed in parallel by different areas of the cortex. Other areas, like V3, V5 and V6, respond primlarily to other stimuli like motion, space etc.
Churchland (21) has noted the many descending axon connections from the visual cortex to the LGN. He emphasises feedback processes in vision and considers recurrent networks as the basis of consciousness. Feedback systems are present also between, for instance, the motion sensitive area V5 and V1 of neural zones related to vision (19). They are also involved in other aspects of the human sensory system such as hearing as witnessed by the role of ciliar neurons in reinforcing an acoustic stimulus in the human cochlea (22).
(d)Newton was the first to discover colours within daylight with his famous prism experiment. He developed the corpuscular light theory which establishes a mathematical basis for light and vision.
(e) The reader is referred to Hundert (23), who characterises interactionism in terms of intersubjectivity, and to Wegner (24), who has re-opened recently the important debate on interactivity in computing which has interesting implications for research in cognitive science. Computer science is founded on the concept of computability as captured by the Turing machine, a closed system. But our present-day machines are interactive, and therefore open systems to which the Church-Turing thesis cannot readily apply. Their computational power is far greater than the theory of computable functions allows. By analogy, in cognitive science, cognition and consciousness can be considered the result not of a single subject perceiving the world but of multiple subjects interacting among themselves and the world.
(f) RGB: The red-green-blue colour code of TV and computer monitors.
(g) The Land algorithm has a reset mechanism which, if a lighter area is encountered during the computation of a path, forces the cumulated relative reflectance down to 0 and restarts the average computation from this area. The effect of the reset mechanism is to consider the lightest area of an image as the reference value of the colour white.
(h) Brownian motion : Erratic random movements performed by microscopic particles in a disperse phase, for instance, particles in suspension in liquid. A brownian path can be seen as an approximation toward the fractal geometry which is typical of many natural structures.
(i) Heisenberg writes "Nature subtracts itself from an accurate description in our intuitive concepts, for the unavoidable perturbation linked to any observation. While the original purpose of any scientific investigation was to describe the nature as it is in itself, without our intervention and observation, now we understand that this purpose is unreachable. In atomic physics it is not possible to abstract in any way from the modifications that are induced in the observed object by any observation. Only the kind of observation establishes what aspects of nature are determined and what are obscured by our observations".
References
1. Itten J. Kunst der Farbe. Otto Meier Verlag, Ravensburg,1970.
2. Hardin CL. Color and illusion. in: Mind and Cognition. A Reader (Lycan WG. Ed) Basil Blackwell, Oxford, pp 555-567,1990.
3. Husserl E. Logische Untersuchungen. Halle (1990-1991)
4. Empedocles. Peri fuseos. In: Grande antologia filosofica. C. Marzorati Ed, Milano, 1954.
5. Plato. Timeo, Laterza, trad. it. C. Giarratano, Bari, 1950.
6. Democrites. Frammenti. In: Grande antologia filosofica, C. Marzorati Ed., Milano,1954.
7. Aristotle. Ibid.
8. Descartes R. Meteore. In: Opere scientifiche, a cura di E. Lojacono, UTET, Torino,1966-83.
9. Goethe JW. Farbenlehre (Pawlik J. Ed.) II edition, DuMont Buchverlag, Köln,1978. Trad. it. Il Saggiatore, Milano, 1979.
10. Newton I. Opticks: or a Treatise of the Reflections, Refractions, Inflections and Colours of Light. IV Ed. London (1730), Dover Pub. Inc., New York (1979)
11. Heisenberg W. Die Farbenlehre in Goethe und Newton. In:Wandlungen in der Grundlagen der Naturwissenschaften, trad. it., Einaudi, Torino, 1965.
12. Sacks O, Wassermann R. The case of the colorblind patient. The New York Review of Books, vol.34, pp 25-34,1987.
13. von Helmholtz H. Optique physiologique. Edition Jacques Gabay, Paris, trad. par Javal E. et Klein N.Th.,1989.
14. Hering E. Beitrag zur Lehre vom Simultankontrast. Zeitschrift fuer Psychologie und Physiologie der Sinnesorgane 1, 18-28,1890.
15. Wyszecky G, Stiles WS. Color science: Concepts and methods, quantitative data and formulas. Wiley, New York, 1982.
16. Marr D. Vision. Freeman, San Francisco,1982.
17. Land E, McCann J. Lightness and Retinex Theory. Journal of Optical Society of America 61(1) 1-11,1971.
18. Land E. The Retinex theory of color vision. Scientific American 237(3) 2-17 (1977)
19. Zeki S. A vision of the brain. Blackwell, Oxford,1994.
20. Hubel D. Eye, brain and vision. Freeman, New York,1988.
21. Churchland P. The engine of reason: the seat of the soul. MIT Press, Cambridge MA, 1995.
22. Roederer JG. The physics and psychophysics of music. Springer Verlag, New York, 1995.
23. Hundert EM. Lessons from an optical illusion. Harvard Univ. Press, Cambridge MA, 1995.
24. Wegner R Interactive foundations of computing. Communications of the ACM, May 1997.
Сайт создан в системе
uCoz